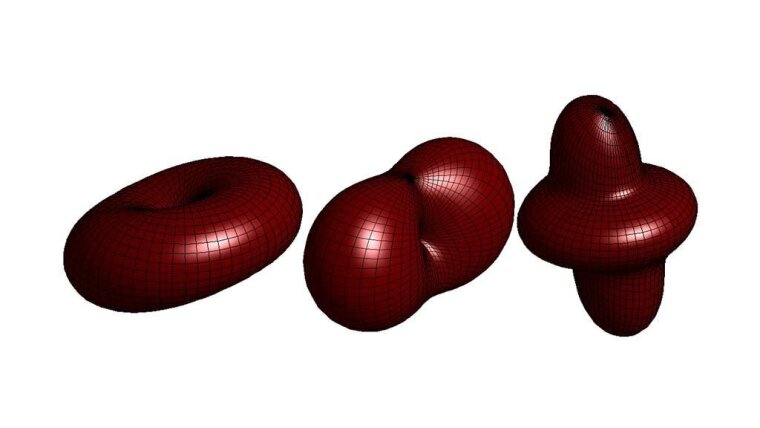
The group studies the interaction of laser light and matter at intensities above the limit that can be described by traditional nonlinear optics. The intensity is too high for the power series expansion of nonlinear optics to converge, but still low enough for quantum coherences to prevail. This field of research, which is called strong field physics or extreme nonlinear optics, leads to phenomena that occur on the attosecond time scale, and at the same time offers the possibility to generate light bursts of attosecond duration. Scientific findings on ultrafast physics and the technological development of ultrafast light sources go hand in hand.
In recent years, promising progress has been made in strong-field light matter interaction in solid materials. Due to their higher density, the generation of high-order harmonics (HHG) from solids could surpass gas-phase sources in terms of conversion efficiency. In addition, the required intensity for HHG from solids is much lower compared to the gas-phase, thus easing the demands on the drive laser. Together with the avoidance of the vacuum beam lines required for gas-phase HHG, solid-state attosecond technology could be applied more widely with simpler and more compact experimental setups.
One goal of the group is to develop new methods of laser pulse generation. Up to now, laser pulses with a duration of one femtosecond or shorter could only be generated in the Vis-IR as well as in the extreme UV, but the deep UV is a spectral range in which such extremely short laser pulses were not available before. Harmonic concatenation is our new method for the synthesis of ultrashort pulses, which delivers record short deep UV waveforms of only 1.5 fs.
Another goal is to understand the physics on the electronic time scale by attosecond spectroscopy. For the manipulation of pump and probe pulses in the Vis-IR and extreme UV, beamlines of several meters in length are normally required. We have developed beamlines on a centimeter scale which facilitate transient absorption and dispersion in the deep UV range. The aim of this project is to measure the nonlinear reaction time of thin glasses, crystals and liquid films to explore the limits of light-induced signal processing.